Methane Pyrolysis
One promising avenue in this pursuit is methane pyrolysis, which holds the key to sustainably producing green hydrogen. In recent years, the world has witnessed an increased urgency to transition towards sustainable, renewable, clean n green energy sources. With concerns over climate change and the finite nature of fossil fuels, scientists and researchers are exploring innovative methods to harness clean n green energy. In this blog, we delve into the fascinating world of methane pyrolysis, exploring its potential, benefits, and challenges in the quest for a clean n green future.
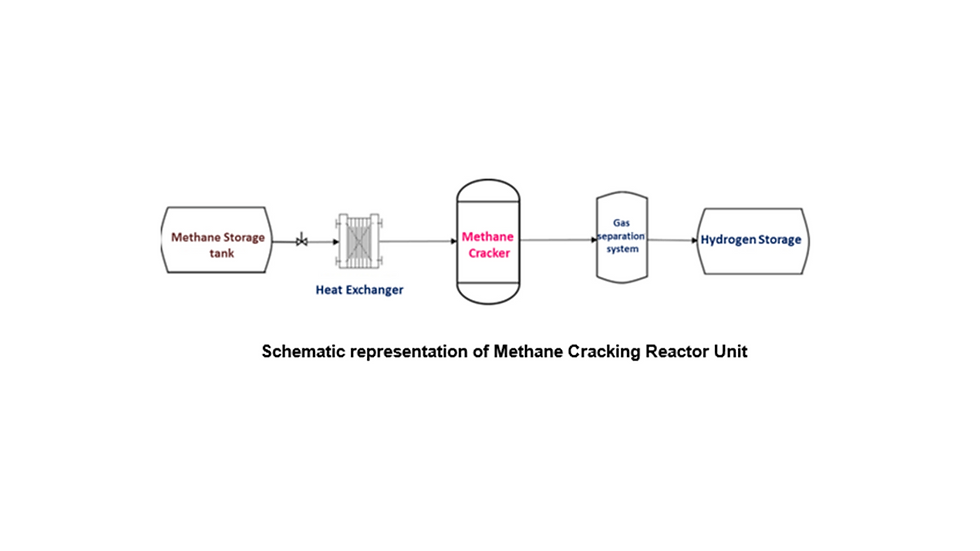
Methane Pyrolysis ?
Methane pyrolysis, also known as methane cracking or methane splitting, is a thermochemical process that utilizes high temperatures to break down methane (CH4) into its two primary components - hydrogen (H2) and solid carbon (C). The reaction can be represented as follows:
CH4 → H2 + C
The process requires temperatures typically ranging from 700°C to 1200°C and can be performed in the presence or absence of a catalyst. Although the concept of methane pyrolysis is not entirely new, recent advancements in materials science and reactor design have made it more viable and cost-effective than ever before.
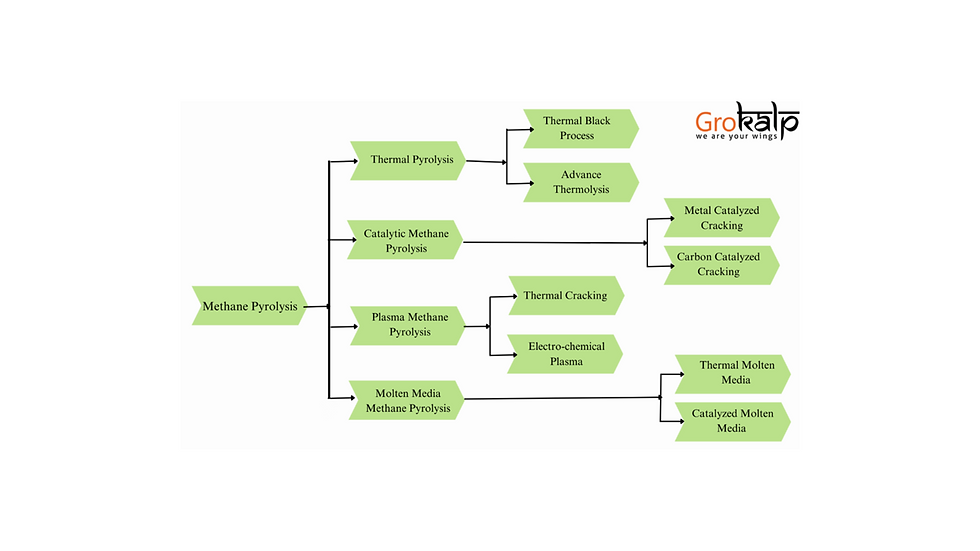
Different technologies' routes of methane pyrolysis
Thermal Methane Pyrolysis:
Thermal methane pyrolysis is the most straightforward and commonly studied route for methane decomposition. It involves subjecting methane to high temperatures (typically between 700°C to 1200°C) in the absence of oxygen, leading to the production of hydrogen and solid carbon. The process can be carried out in different types of reactors, including fixed-bed reactors, fluidized bed reactors, or even solar-driven reactors. However, one of the main challenges of thermal pyrolysis is the high energy input required to reach the necessary temperatures, which can impact the overall efficiency and cost-effectiveness of the process.
Plasma Methane Pyrolysis:
Plasma methane pyrolysis involves utilizing plasma, an ionized gas consisting of highly energized particles, to decompose methane. The plasma is generated by applying a high-voltage electric discharge to a gas, creating a high-energy environment where methane molecules can be dissociated into hydrogen and carbon. Plasma pyrolysis offers advantages such as rapid reaction rates, precise control of process conditions, and the possibility of using a wider range of feedstocks. However, the technology is still in its early stages of development, and scaling up plasma reactors while maintaining cost-effectiveness remains a challenge.
Catalytic Methane Pyrolysis:
Catalytic methane pyrolysis involves the use of catalysts to facilitate the methane decomposition reaction at lower temperatures and reduce the energy requirements. The catalysts help break the methane bonds more effectively, thereby enabling the process to occur at milder conditions compared to thermal pyrolysis. This technology route has the potential to enhance process efficiency and reduce energy consumption significantly. Research is ongoing to identify and develop suitable catalyst materials that offer high activity, stability, and selectivity for the desired products. However, finding cost-effective and durable catalysts remains a key challenge for commercial implementation
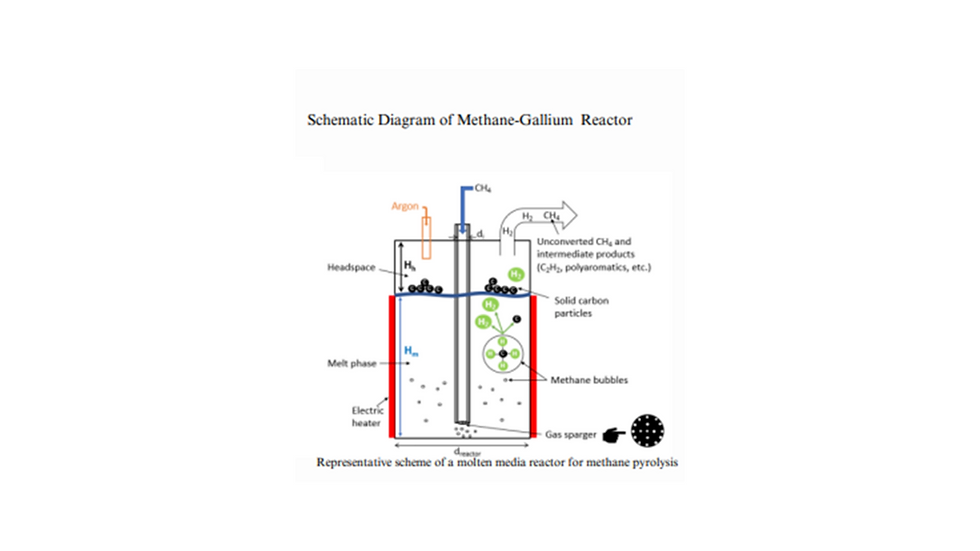
Molten media methane pyrolysis:
Molten media methane pyrolysis is an innovative and promising approach for the decomposition of methane. In this method, methane is reacted in the presence of a molten metal or molten salt, which serves as both the reaction medium and a heat source. The molten media enhances the heat transfer, enabling efficient thermal decomposition of methane at lower temperatures compared to traditional thermal pyrolysis. This process offers the potential for increased energy efficiency and reduced carbon formation, making it a compelling avenue for sustainable hydrogen production and carbon utilization, contributing to a cleaner and more sustainable future.
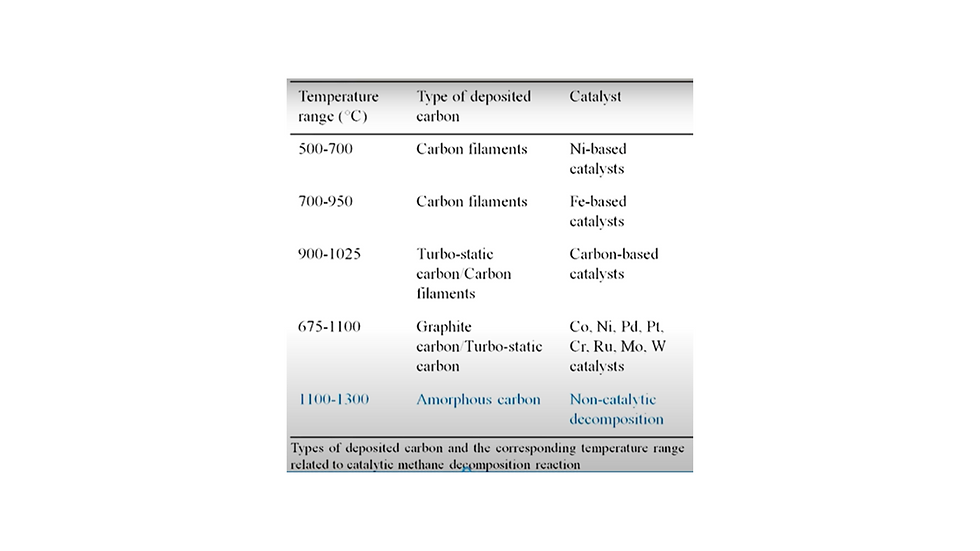
Catalysts used for methane pyrolysis:
Nickel-Based Catalysts:
Nickel-based catalysts are among the most common and widely studied catalysts for methane pyrolysis. These catalysts exhibit good activity for the reaction and can operate at moderate temperatures. They facilitate the dissociation of methane into hydrogen and solid carbon while minimizing carbon formation and catalyst deactivation. However, nickel catalysts may require higher temperatures to achieve high conversion rates, and they are susceptible to deactivation over time due to carbon deposition on the catalyst surface.
Supported Metal Catalysts:
Supported metal catalysts involve depositing catalytically active metals, such as nickel, cobalt, or iron, onto a high-surface-area support material, typically alumina, silica, or zeolites. These catalysts offer better stability and selectivity compared to pure metal catalysts. They can be tailored to control the reaction kinetics and improve the production of hydrogen while minimizing undesirable carbon formation.
Noble Metal Catalysts:
Noble metal catalysts, such as platinum and palladium, have shown high catalytic activity for methane pyrolysis. These catalysts can operate at relatively lower temperatures and exhibit excellent selectivity towards hydrogen production. However, noble metals are expensive, limiting their large-scale commercial applications.
Bimetallic Catalysts:
Bimetallic catalysts combine two different metal components, often with a promoter, to synergistically enhance catalytic activity and stability. For example, a combination of nickel and cobalt has been investigated as a bimetallic catalyst for methane pyrolysis, resulting in improved catalytic performance and reduced carbon deposition.
Perovskite-Type Oxide Catalysts:
Perovskite-type oxide catalysts, such as LaAlO3 and LaCoO3, have shown promising results in methane pyrolysis. These catalysts possess high oxygen mobility, promoting the redox reaction required for methane dissociation. They can operate at moderate temperatures and exhibit good stability during the reaction.
Non-Metallic Catalysts:
Non-metallic catalysts, including transition metal carbides, nitrides, and oxides, have gained attention for methane pyrolysis. For example, molybdenum carbide has shown excellent catalytic activity and stability for the reaction, offering a potential alternative to traditional metal catalysts.
The choice of catalyst for methane pyrolysis depends on factors such as reaction conditions, desired product selectivity, and economic considerations. Researchers continue to explore and develop new catalysts to enhance the efficiency and sustainability of methane pyrolysis, making it a viable route for hydrogen production and carbon utilization in the quest for cleaner energy solutions.
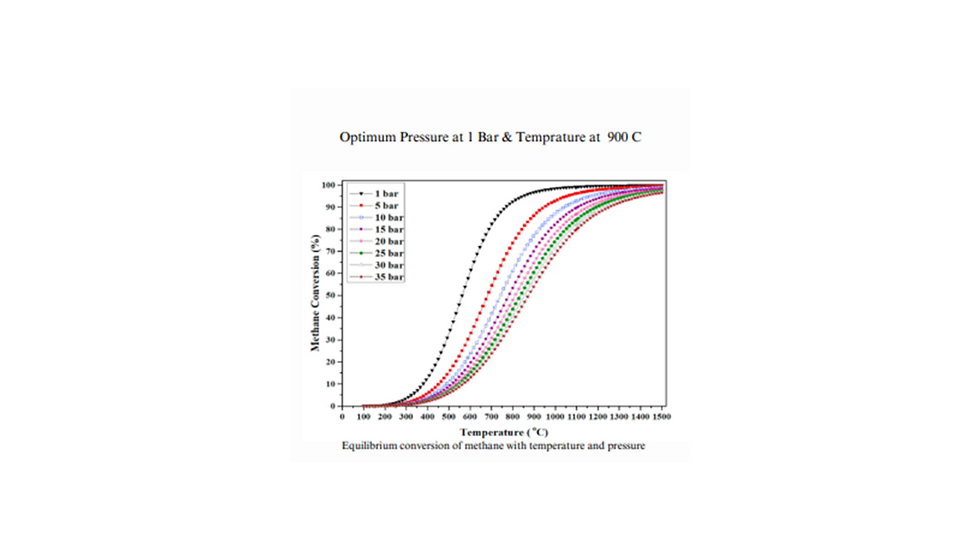
The equilibrium conversion of methane is significantly influenced by temperature and pressure in chemical reactions, including methane pyrolysis. As an endothermic reaction, the forward decomposition of methane into hydrogen and solid carbon is favored at higher temperatures. Increasing the temperature shifts the equilibrium towards the products, leading to higher methane conversion rates. On the other hand, pressure has a limited effect on the equilibrium conversion due to the balanced stoichiometry of the reaction. While changes in pressure can influence reaction kinetics and reactor performance, it does not significantly alter the position of the equilibrium. Achieving the desired equilibrium conversion of methane requires careful optimization of temperature, pressure, and other reaction parameters to maximize the production of valuable products while considering practical and economic constraints
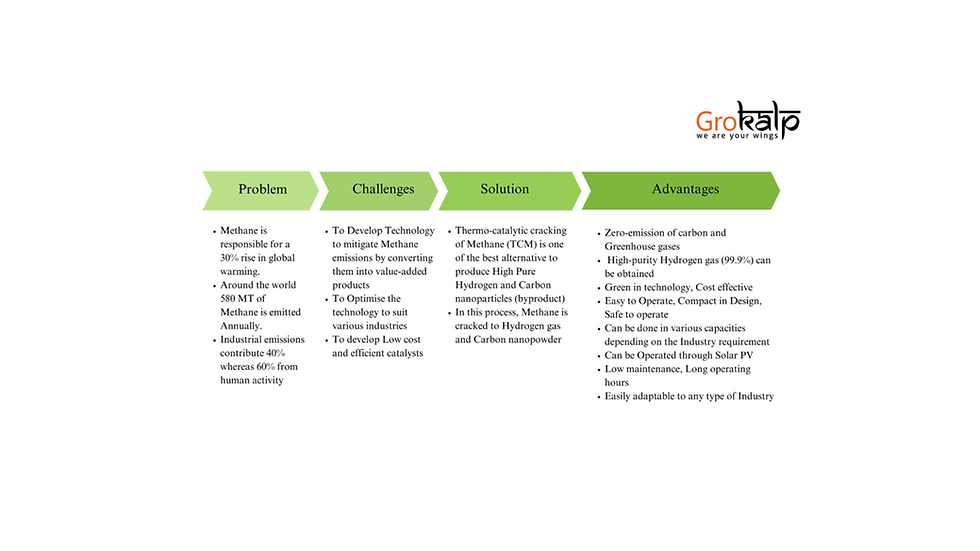
Key Advantages of Methane Pyrolysis
Green Hydrogen Production: Hydrogen generated through methane pyrolysis is considered "green hydrogen" since it is produced without emitting CO2. This aligns with global efforts to reduce greenhouse gas emissions and combat climate change.
Carbon Capture and Utilization (CCU): The solid carbon produced during methane pyrolysis can find applications in various industries, such as construction materials or energy storage. Utilizing this solid carbon provides a viable method of carbon capture and utilization, contributing to a circular carbon economy.
Utilization of Natural Gas Resources: As methane is the primary component of natural gas, methane pyrolysis allows for the efficient utilization of existing natural gas infrastructure. This enables a smoother transition towards renewable energy sources while leveraging existing resources.
Potential for Energy Storage: The solid carbon produced during methane pyrolysis can act as a form of energy storage, releasing the stored energy when needed through combustion or other processes.
Challenges and Roadblocks
While methane pyrolysis holds tremendous promise, there are several challenges that researchers and engineers must address:
Energy Input: The process requires a substantial amount of energy input to reach the necessary temperatures for methane cracking. Finding cost-effective and sustainable energy sources is critical to make the process economically viable.
Catalyst Development: Identifying efficient and durable catalysts that facilitate the pyrolysis reaction is essential for optimizing the process and reducing energy requirements.
Scale-up and Commercialization: While laboratory-scale demonstrations have shown promise, scaling up methane pyrolysis to industrial levels presents engineering and economic challenges.
Infrastructure Adaptation: Adapting existing natural gas infrastructure for methane pyrolysis may require substantial investments and modifications.
Conclusion
Methane pyrolysis represents a compelling avenue for green hydrogen production and carbon capture. As the world races towards a sustainable future, this technology offers the potential to decarbonize multiple sectors, reduce greenhouse gas emissions, and utilize existing natural gas resources more efficiently.
As researchers continue to innovate and overcome the challenges associated with methane pyrolysis, this process could play a pivotal role in shaping a cleaner and greener energy landscape for generations to come. Embracing such cutting-edge technologies is essential in our collective journey to combat climate change and ensure a sustainable future for our planet.
Comments